Myoelectric prosthesis technology is engineered to read muscle signals and translate them into motorized limb movements, offering users enhanced autonomy. This article will explore how these devices, such as the myoelectric prosthesis, seamlessly integrate with the human body and the cutting-edge innovations that are continuously improving their functionality and user experience.
Key Takeaways
- Myoelectric prostheses operate using electromyographic signals from muscles, allowing users to control prosthetic movements with precision, providing both speed and grip control that aligns with user intentions. Continuous advancements in sensor technology and hand control mechanisms have significantly improved their functionality and user experience.
- Achieving natural and intuitive control over myoelectric prostheses is facilitated by cutting-edge technology such as pattern recognition and targeted muscle reinnervation surgery. Feedback systems are crucial, providing sensory feedback to users and thereby enhancing the usability and comfort of the prosthetic limbs.
- Mastering a myoelectric prosthesis is a comprehensive process that involves extensive training to control muscle contraction and operate the prosthetic device effectively. Challenges such as EMG signal variability and electrode interface issues are addressed through ongoing innovations, while users must face an emotional journey to adapt to and embrace their prosthetic for daily life.
Understanding Myoelectric Prostheses
Myoelectric prostheses represent a remarkable convergence of biology and technology. They operate by harnessing the electrical signals produced by your muscles to regulate the movements of the prosthesis, functioning as a closed loop control system. These prosthetic devices utilize electromyographic (EMG) signals produced in skeletal muscles, captured by electrodes positioned on the skin surface or inserted in the remaining limb.
Over the years, myoelectric prostheses, especially upper limb prosthetics, have undergone significant evolution. From the introduction of the first clinically significant myoelectric prosthesis in 1960 that incorporated transistors to enhance functionality and reduce bulk, to present-day advancements like myoelectric sensors, the journey has been transformative.
The Science Behind Myoelectric Control
A deeper look into the science of myoelectric control involves understanding that it functions based on electromyographic (EMG) signals generated by skeletal muscles. These signals are captured by surface electrodes and sent to the prosthetic devices for regulating their motions. This form of control enables users to have precise influence over the speed of finger movement and the rate at which gripping force intensifies, aligning with the user’s intentions. Tactile feedback is an essential aspect of myoelectric prosthetics, as it helps users better control the prosthesis.
Sensors are a fundamental component in myoelectric prosthetics. Devices like implantable myoelectric sensors detect and transmit electromyographic (EMG) signals from the contracted muscles in the residual limb. These signals are vital for the accurate operation of the myoelectric prosthetic device, thereby improving its functionality and user control.
Advancements in Prosthetic Hand Control
Prosthetic hand control has undergone substantial advancements throughout the years. One major breakthrough is the development of multi-grip myoelectric prosthetic hand devices. These devices are equipped with five movable and jointed fingers, allowing for a wide range of grip patterns that closely resemble the natural movement of a human hand, significantly enhancing functionality.
Another significant development is the creation of a new bionic hand that allows precise control over each finger, significantly improving the user’s ability to make precise and varied movements with their prosthetic arm. Moreover, the adjustable grip force feature offers users the ability to customize the force applied to objects, facilitating precise tasks and enabling them to manipulate a wide range of objects effortlessly.
Types of Myoelectric Prostheses
The market offers a variety of myoelectric prostheses, including:
- Hand components
- Wrist components
- Elbow components Renowned models include:
- iLimb
- Vincent Hand
- iLimb Pulse
- SensorHand
- BeBionic
These devices rely on signal processing techniques to interpret and utilize the EMG signals generated by the user’s muscles.
The basic design of a single grip terminal myoelectric prosthesis enables the opening and closing of one grip position, providing users with essential gripping functionality. However, more advanced myoelectric prostheses are equipped with:
- More intuitive control
- Increased grip strength
- Multiple grip patterns
- The capacity for complex movements
These devices greatly improve the performance and satisfaction in daily activities by enabling users to customize their grip for individual tasks, resulting in more efficient and comfortable interaction with their environment.
Achieving Natural Control Through Technology
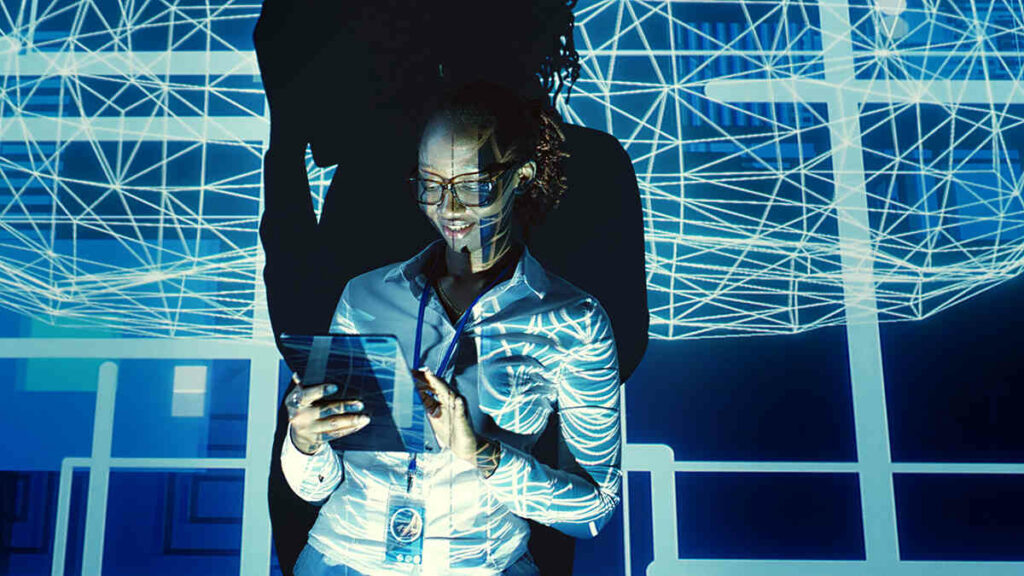
Innovative technology significantly contributes to achieving a more natural and intuitive control of myoelectric prostheses. Pattern recognition is one such technology, which involves the application of machine learning methods to categorize and interpret EMG signals generated by residual limb muscles. This enables users to command the prosthesis through their muscle contractions.
Feedback systems are integral in improving the usability of myoelectric prostheses. They provide essential sensory feedback, which is greatly valued by prosthesis users. This feedback plays a crucial role in tasks like grasping and enhances the overall ease of use and body image of the prosthesis, offering a more seamless user experience.
Intuitive Control Mechanisms
Intuitive control mechanisms, like targeted muscle reinnervation (TMR), are revolutionizing the way amputees operate prosthetic limbs. TMR is a surgical technique that improves the control of upper limb prostheses by allowing individuals to utilize residual nerves from the amputated limb, which are redirected to different muscle sites.
This innovative approach allows users to:
- Manipulate the prosthetic limb in a more natural and intuitive manner
- Enhance both control and sensory feedback in myoelectric prosthetic hands
- Improve the user’s ability to perform a diverse range of movements without the need for frequent switching.
Feedback Systems Enhancing Usability
Feedback systems play a crucial role in improving the usability of myoelectric prostheses. They provide crucial sensory feedback, which is greatly valued by prosthesis users. This feedback plays a significant role in tasks like grasping and enhances the overall comfort and usability of the prosthesis.
Various types of feedback systems are employed in myoelectric prostheses, such as:
- Supplementary sensory feedback
- Force sensing
- Temperature sensing
- Motion sensing
- Augmented reality feedback
Augmented reality feedback, in particular, strengthens the internal model, improves prosthesis control performance, and provides valuable auditory feedback.
Mastering the Use of a Myoelectric Prosthesis
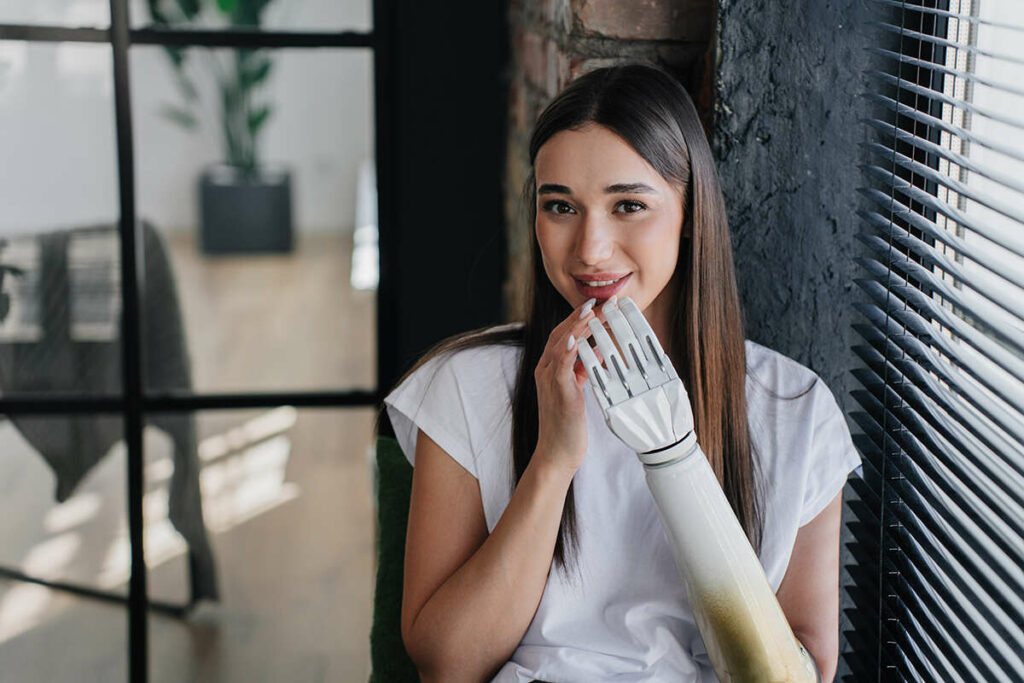
Mastering the use of a myoelectric prosthesis requires comprehensive training. It involves acquiring the skill to consciously control muscle contraction and developing the capability to operate the prosthetic hand. The training process for using a myoelectric prosthesis includes:
- Programming the prosthesis controller
- Recording EMG signals
- Developing conscious control over muscle contraction
- Engaging in daily training sessions lasting 1 to 2 hours.
Physical preparation is another crucial aspect, involving:
- Enhancement of muscle strength
- Guidance on limb hygiene
- Promotion of independence
- Myoelectric site testing to ensure optimal functioning of the prosthesis.
Training for Optimal Functionality
Achieving optimal functionality with myoelectric prostheses necessitates thorough training. It includes preprosthetic physical and signals training, integration, and control training. This training is vital to ensure that users can effectively utilize their prosthesis and maximize its various functions in daily activities, resulting in improved short-term and long-term prosthetic performance.
Signals training involves instructing individuals on how to manipulate the prosthetic device by utilizing the electrical signals produced by their muscles. These signals are usually captured through surface electrodes positioned on the skin over specific muscles or muscle groups. Integration training can encompass a range of methods, including pattern recognition training, and the use of training devices tailored for upper limb prostheses.
Role of Visual and Augmented Reality Feedback
Visual and augmented reality feedback systems are instrumental in training users to control their myoelectric prostheses effectively. Some benefits of using visual feedback include:
- Enhancement of myoelectric prostheses
- Improved performance time
- Increased accuracy
- Enhanced usability
Augmented reality has a significant impact on the training of myoelectric prosthesis users by offering a modality for training control of the prosthesis. It has the potential to enhance motivation and improve training effectiveness.
These systems utilize augmented reality (AR) to deliver visual feedback, allowing participants to interact with a virtual prosthesis and manipulate virtual objects, thereby enhancing their motivation and engagement during the training.
Challenges and Solutions in Myoelectric Control
Despite the remarkable functionality of myoelectric prostheses, they pose some challenges. Key among these is EMG signal variability, which can lead to instability in pattern recognition control of myoelectric prostheses and can also affect the amplitude of the EMG signal used to control the movements of the artificial limb.
Another challenge lies in the electrode interface in myoelectric prostheses, which may pose challenges including:
- Undesired prosthesis closure
- External interferences
- Changes in electrode impedance
- Muscle weakness
- Electrode movement
- Evolving skin impedance
- Day-to-day variability in electrode placement
Despite these challenges, innovations are continuously being made to address these issues and enhance the functionality of myoelectric prostheses.
Understanding EMG Signal Variability
In the context of myoelectric prostheses, EMG signal variability refers to the non-stationary nature of EMG signals in real-life scenarios, impacting the stability of pattern recognition control. Addressing this variability is crucial to ensure the provision of more dependable information for the prosthetic device.
The variability of EMG signals in myoelectric prostheses can be attributed to:
- The non-stationary nature of EMG signals
- Intensity variability
- Disturbances such as motion artifacts
- Abnormal co-contraction features in the residual limb
- The order of recruitment of motor units
EMG signal variability has a direct impact on the amplitude of the signal and can lead to instability in myoelectric pattern recognition control.
Techniques utilized to reduce EMG signal variability in myoelectric prostheses involve capturing signals from specific muscle groups and implementing decoding methods like proportional control.
Innovations in Electrode Interface Design
Another hurdle to overcome is the challenges with the electrode interface in myoelectric prostheses. Innovations in electrode interface design aim to reduce unpredictability by ensuring stable electrode contact with the skin for reliable EMG signal transduction.
To improve signal transduction, designs for myoelectric prostheses are now integrating:
- Motosensory devices into the implant
- Intelligent interfaces for interpreting muscle electrical activity to suggest movement
- Utilization of RPNIs and implanted EMG electrodes for improved prosthetic control
- Implanted transducers for enhanced kinesthetic communication.
Improved electrode interface design reduces unpredictability by ensuring stable electrode contact with the skin for reliable EMG signal transduction.
Real-Life Impact: Stories from Myoelectric Prosthesis Users
The true measure of the impact of myoelectric prostheses is best seen through the experiences of those who use them. These devices have transformed the lives of numerous individuals, enabling them to:
- Regain their independence
- Engage in day-to-day activities
- Improve their self-esteem
- Enhance their quality of life
Across the globe, there are countless stories of people overcoming physical challenges with the help of myoelectric prostheses. From performing basic daily tasks to engaging in hobbies requiring dexterity, these devices have proven invaluable, paving the way for everyday triumphs.
Everyday Triumphs with Myoelectric Control
Everyday triumphs with myoelectric control come in many forms. For some, it’s the ability to perform daily activities with ease and improved self-esteem, while for others it’s the sense of normalcy and the restoration of quality of life.
The utilization of myoelectric prosthetics has a substantial influence on daily life, enhancing the execution of daily activities and overall contentment with personally selected activities of daily living, including hobbies and tasks requiring dexterity. The utilization of a myoelectric prosthesis can contribute to:
- The improvement of self-esteem and overall quality of life through enhanced functionality
- Increased strength and grip ability
- Sensory feedback
- Improved social interactions and body image.
The Emotional Journey
The journey of using a myoelectric prosthesis can be an emotional one. Users often face challenges in controlling the device, which can result in passive use or rejection. They commonly face a variety of challenges including:
- Mental and physical demands
- Visual demands
- Need for conscious processing
- Frustration
- Situational stress
- Time pressure
- Device-related issues
However, with support and training, users can overcome these challenges. The embodiment of the prosthesis gradually diminishes the impact of environmental barriers, resulting in enhanced autonomy and confidence. This process is often accompanied by a sense of achievement, as users conquer each challenge and gain increased independence and control over their lives.
Selecting the Right Myoelectric Prosthesis for You
Selecting the right myoelectric prosthesis involves considering a range of personal factors, such as:
- Physical abilities
- Activities and participation
- Mental well-being
- Social factors
- Rehabilitation needs
- Personal preferences
- Prosthetic experience
- Functional needs
- Control schemes
- Amputation level
- Residual limb geometry
- Sensation
This is a key step towards regaining independence and functionality through improved task performance.
The selection of a myoelectric prosthesis is significantly influenced by an individual’s abilities, specific needs, and personal goals, including their functional needs, control preferences, and the desired level of prosthetic use. Because of the high rejection rates of upper limb prostheses, it’s crucial for upper limb prosthesis users to promptly address challenges with prosthesis control and seek assistance from healthcare professionals to effectively overcome obstacles and avoid discontinuation of device usage.
Factors to Consider
Several key factors should be considered when selecting a myoelectric prosthesis. The level of amputation, for instance, influences the available prosthetic options, which encompass:
- passive
- body-powered
- myoelectric
- activity-specific
- hybrid systems
Your desired functionality is another important consideration. This includes:
- Your physical abilities
- Activities and participation
- Mental well-being
- Social interactions
- Rehabilitation needs
- Personal preferences
- Prosthetic experience
- Musculoskeletal integrity
- And more
Other factors to consider include the appearance of the prosthesis, which can influence your satisfaction with cosmetic appearance and psychosocial and social adaptation, and the funding options available for myoelectric prostheses.
Consulting with Certified Professionals
Due to the complexity of choosing the right myoelectric prosthesis, consulting with certified professionals is highly recommended. Prosthetists conduct the following tasks:
- Patient assessments
- Devising, measuring, and properly fitting the prostheses
- Determining the residual limb muscles that are most effective in controlling the prosthesis through their electrical signals.
When selecting a prosthetist, ensure they:
- Hold certification from the American Board for Certification
- Possess three to five years of upper limb experience
- Demonstrate professionalism, knowledge, reliability, and national certification, along with any necessary licensing
They will guide you through the selection process, providing tailored advice and suggestions based on your unique needs and goals.
Innovations in Myoelectric Prosthesis Research
Myoelectric prosthesis research is driven by innovation. Researchers are focusing on breakthroughs in sensory feedback and future directions in prosthetic development to enhance user experience and control.
The use of AI and robotics in the design of myoelectric prostheses is also influencing the future of this field. These advancements aim to provide:
- More intuitive control
- Improved grip strength
- Diverse gripping patterns
- Potential enhancements in sensory feedback for users.
Breakthroughs in Sensory Feedback
Recent breakthroughs in sensory feedback for myoelectric prostheses have been remarkable. Researchers have developed a hybrid multi-modal sensory feedback system that provides non-invasive sensory feedback through:
- Haptic feedback
- Proprioceptive feedback
- Thermal feedback
- Vibrotactile feedback
This system has been shown to improve the performance of prosthetic hands over both short-term and extended use.
Sensory feedback contributes to improving the user experience in myoelectric prostheses by:
- Facilitating easier use
- Improving body image
- Enhancing social interaction
- Increasing willingness to wear and use the prosthesis
- Aiding in the performance of grasping tasks
Future Directions in Prosthetic Development
With the integration of advanced technologies and strategies to enhance control, the future of prosthetic development looks promising. Some examples of how technology is shaping the development of myoelectric prostheses include:
- Game-based training
- Myoelectric control-based prosthetic hands
- Intuitive and intelligent myoelectric control
- Vibrotactile feedback
These advancements are paving the way for more functional and natural prosthetic limbs, including the development of body powered prosthesis options.
However, the path to advanced prosthetic development isn’t without challenges. Some of the anticipated hurdles in the future development of myoelectric prosthetics include:
- Enhancing biocompatibility with the human body
- Tackling the existing status and challenges of upper extremity prosthetics
- Investigating the potential of virtual environments in prosthetic systems
These challenges will need to be addressed in order to continue advancing prosthetic technology.
Summary
From understanding the science behind myoelectric prostheses to appreciating the real-life impact these devices have on users, we’ve delved into the intricacies of this transformative technology. Myoelectric prostheses have come a long way from their inception, providing a new lease of life for individuals with limb amputations. With continued research and development, the future holds exciting possibilities for further enhancements in functionality, control, and user experience. As we wrap up, remember that the journey with a myoelectric prosthesis is one of resilience, adaptation, and triumph.
Frequently Asked Questions
What is the difference between mechanical and myoelectric prosthesis?
The main difference between mechanical and myoelectric prostheses is in how they are operated: mechanical prostheses use cables and a body harness, while myoelectric prostheses use electrical motors and skin surface electrodes. Choose the type that best fits your needs.
How much does a myoelectric hand prosthesis cost?
A myoelectric hand prosthesis can cost anywhere from $15,000 to $100,000, making it a significant investment. Repairs typically require expensive proprietary components and professional assistance.
What are the four types of prosthetics?
The four types of prosthetics are transradial, transhumeral, transtibial, and transfemoral. Each type serves a different function depending on the missing body part.
What is the science behind myoelectric prostheses?
Myoelectric prostheses work by using the electrical signals from your muscles to control the prosthesis movements through electromyographic (EMG) signals. These signals are captured by electrodes placed on the skin or inserted in the remaining limb.
How has prosthetic hand control evolved?
Prosthetic hand control has evolved significantly over the years, with advancements such as multi-grip functions, individual finger mobility, and adjustable grip force, greatly improving the user experience.
Leave a Reply
You must be logged in to post a comment.